Relativity of Mankind - Einstein and his Time 
(contenuti)
0. Starter Module – Einstein’s life
I never think of the future -- it will come soon enough.
Albert
Einstein
Born: 14 March 1879 in Ulm, Württemberg, Germany - Died: 18 April 1955
in Princeton, New Jersey, USA
Around 1886 Albert Einstein began his school career in Munich. As well as
his violin lessons, which he had from age six to age thirteen, he also had
religious education at home where he was taught Judaism. Two years later he
entered the Luitpold Gymnasium and after this his religious education was
given at school. He studied mathematics, in particular the calculus,
beginning around 1891.
In 1894 Einstein’s family moved to Milan but Einstein remained in Munich.
In 1895 Einstein failed an examination that would have allowed him to study
for a diploma as an electrical engineer at the Eidgenössische Technische
Hochschule in Zurich. Einstein renounced German citizenship in 1896 and was
to be stateless for a number of years. He did not even apply for Swiss
citizenship until 1899, citizenship being granted in 1901.
Following the failing of the entrance exam to the ETH, Einstein attended
secondary school at Aarau planning to use this route to enter the ETH in
Zurich. While at Aarau he wrote an essay (for which was only given a little
above half marks!) in which he wrote of his plans for the future:-
If I were to have the good fortune to pass my examinations, I would go
to Zurich. I would stay there for four years in order to study mathematics
and physics. I imagine myself becoming a teacher in those branches of the
natural sciences, choosing the theoretical part of them. Here are the
reasons which lead me to this plan. Above all, it is my disposition for
abstract and mathematical thought, and my lack of imagination and practical
ability.
Indeed Einstein succeeded with his plan graduating in 1900 as a teacher
of mathematics and physics. One of his friends at ETH was Marcel
Grossmann who was in the same class as Einstein. Einstein tried to
obtain a post, writing to Hurwitz who held out some hope of a position but
nothing came of it. Three of Einstein’s fellow students, including
Grossmann, were appointed assistants at ETH in Zurich but clearly Einstein
had not impressed enough and still in 1901 he was writing round universities
in the hope of obtaining a job, but without success.
He did manage to avoid Swiss military service on the grounds that he had
flat feet and varicose veins. By mid 1901 he had a temporary job as a
teacher, teaching mathematics at the Technical High School in Winterthur.
Around this time he wrote:-
I have given up the ambition to get to a university ...
Another temporary position teaching in a private school in Schaffhausen
followed. Then Grossmann’s father tried to help Einstein get a job
by recommending him to the director of the patent office in Bern. Einstein
was appointed as a technical expert third class.
Einstein worked in this patent office from 1902 to 1909, holding a temporary
post when he was first appointed, but by 1904 the position was made
permanent and in 1906 he was promoted to technical expert second class.
While in the Bern patent office he completed an astonishing range of
theoretical physics publications, written in his spare time without the
benefit of close contact with scientific literature or colleagues.
Einstein earned a doctorate from the University of Zurich in 1905 for a
thesis On a new determination of molecular dimensions. He dedicated the
thesis to Grossmann.
In the first of three papers, all written in 1905, Einstein examined the
phenomenon discovered by Max Planck, according to which electromagnetic
energy seemed to be emitted from radiating objects in discrete quantities.
The energy of these quanta was directly proportional to the frequency of the
radiation. This seemed to contradict classical electromagnetic theory, based
on Maxwell’s equations and the laws of thermodynamics which assumed that
electromagnetic energy consisted of waves which could contain any small
amount of energy. Einstein used Planck’s quantum hypothesis to describe
the electromagnetic radiation of light.
Einstein’s second 1905 paper proposed what is today called the special
theory of relativity. He based his new theory on a reinterpretation of the
classical principle of relativity, namely that the laws of physics had to
have the same form in any frame of reference. As a second fundamental
hypothesis, Einstein assumed that the speed of light remained constant in
all frames of reference, as required by Maxwell’s theory.
Later in 1905 Einstein showed how mass and energy were equivalent. Einstein
was not the first to propose all the components of special theory of
relativity. His contribution is unifying important parts of classical
mechanics and Maxwell’s electrodynamics.
The third of Einstein’s papers of 1905 concerned statistical mechanics, a
field of that had been studied by Ludwig Boltzmann and Josiah Gibbs.
After 1905 Einstein continued working in the areas described above. He made
important contributions to quantum theory, but he sought to extend the
special theory of relativity to phenomena involving acceleration. The key
appeared in 1907 with the principle of equivalence, in which gravitational
acceleration was held to be indistinguishable from acceleration caused by
mechanical forces. Gravitational mass was therefore identical with inertial
mass.
In 1908 Einstein became a lecturer at the University of Bern after
submitting his Habilitation thesis Consequences for the constitution of
radiation following from the energy distribution law of black bodies. The
following year he become professor of physics at the University of Zurich,
having resigned his lectureship at Bern and his job in the patent office in
Bern.
By 1909 Einstein was recognised as a leading scientific thinker and in that
year he resigned from the patent office. He was appointed a full professor
at the Karl-Ferdinand University in Prague in 1911. In fact 1911 was a very
significant year for Einstein since he was able to make preliminary
predictions about how a ray of light from a distant star, passing near the
Sun, would appear to be bent slightly, in the direction of the Sun. This
would be highly significant as it would lead to the first experimental
evidence in favour of Einstein’s theory.
About 1912, Einstein began a new phase of his gravitational research, with
the help of his mathematician friend Marcel Grossmann, by expressing his
work in terms of the tensor calculus of Tullio Levi-Civita and Gregorio
Ricci-Curbastro. Einstein called his new work the general theory of
relativity. He moved from Prague to Zurich in 1912 to take up a chair at the
Eidgenössische Technische Hochschule in Zurich.
Einstein returned to Germany in 1914 but did not reapply for German
citizenship. What he accepted was an impressive offer. It was a research
position in the Prussian Academy of Sciences together with a chair (but no
teaching duties) at the University of Berlin. He was also offered the
directorship of the Kaiser Wilhelm Institute of Physics in Berlin which was
about to be established.
After a number of false starts Einstein published, late in 1915, the
definitive version of general theory. Just before publishing this work he
lectured on general relativity at Göttingen and he wrote:-
To my great joy, I completely succeeded in convincing Hilbert and Klein.
In fact Hilbert submitted for publication, a week before Einstein
completed his work, a paper which contains the correct field equations of
general relativity.
When British eclipse expeditions in 1919 confirmed his predictions, Einstein
was idolised by the popular press. The London Times ran the headline on 7
November 1919:-
Revolution in science - New theory of the Universe - Newtonian ideas
overthrown.
In 1920 Einstein’s lectures in Berlin were disrupted by demonstrations
which, although officially denied, were almost certainly anti-Jewish.
Certainly there were strong feelings expressed against his works during this
period which Einstein replied to in the press quoting Lorentz, Planck and
Eddington as supporting his theories and stating that certain Germans would
have attacked them if he had been:-
... a German national with or without swastika instead of a Jew with
liberal international convictions...
During 1921 Einstein made his first visit to the United States. His main
reason was to raise funds for the planned Hebrew University of Jerusalem.
However he received the Barnard Medal during his visit and lectured several
times on relativity. He is reported to have commented to the chairman at the
lecture he gave in a large hall at Princeton which was overflowing with
people:-
I never realised that so many Americans were interested in tensor
analysis.
Einstein received the Nobel Prize in 1921 but not for relativity rather
for his 1905 work on the photoelectric effect. In fact he was not present in
December 1922 to receive the prize being on a voyage to Japan. Around this
time he made many international visits. He had visited Paris earlier in 1922
and during 1923 he visited Palestine. After making his last major scientific
discovery on the association of waves with matter in 1924 he made further
visits in 1925, this time to South America.
Among further honours which Einstein received were the Copley Medal of the
Royal Society in 1925 and the Gold Medal of the Royal Astronomical Society
in 1926.
Niels Bohr and Einstein were to carry on a debate on quantum theory which
began at the Solvay Conference in 1927. Planck, Niels Bohr, de Broglie,
Heisenberg, Schrödinger and Dirac were at this conference, in addition to
Einstein. Einstein had declined to give a paper at the conference and:-
... said hardly anything beyond presenting a very simple objection to
the probability interpretation .... Then he fell back into silence ...
Indeed Einstein’s life had been hectic and he was to pay the price in
1928 with a physical collapse brought on through overwork. However he made a
full recovery despite having to take things easy throughout 1928.
By 1930 he was making international visits again, back to the United States.
A third visit to the United States in 1932 was followed by the offer of a
post at Princeton. The idea was that Einstein would spend seven months a
year in Berlin, five months at Princeton. Einstein accepted and left Germany
in December 1932 for the United States. The following month the Nazis came
to power in Germany and Einstein was never to return there.
During 1933 Einstein travelled in Europe visiting Oxford, Glasgow, Brussels
and Zurich. Offers of academic posts which he had found it so hard to get in
1901, were plentiful. He received offers from Jerusalem, Leiden, Oxford,
Madrid and Paris.
What was intended only as a visit became a permanent arrangement by 1935
when he applied and was granted permanent residency in the United States. At
Princeton his work attempted to unify the laws of physics. However he was
attempting problems of great depth and he wrote:-
I have locked myself into quite hopeless scientific problems - the more
so since, as an elderly man, I have remained estranged from the society here...
In 1940 Einstein became a citizen of the United States, but chose to
retain his Swiss citizenship. He made many contributions to peace during his
life. In 1944 he made a contribution to the war effort by hand writing his
1905 paper on special relativity and putting it up for auction. It raised
six million dollars, the manuscript today being in the Library of Congress.
By 1949 Einstein was unwell. A spell in hospital helped him recover but he
began to prepare for death by drawing up his will in 1950. He left his
scientific papers to the Hebrew University in Jerusalem, a university which
he had raised funds for on his first visit to the USA, served as a governor
of the university from 1925 to 1928 but he had turned down the offer of a
post in 1933 as he was very critical of its administration.
One more major event was to take place in his life. After the death of the
first president of Israel in 1952, the Israeli government decided to offer
the post of second president to Einstein. He refused but found the offer an
embarrassment since it was hard for him to refuse without causing offence.
One week before his death Einstein signed his last letter. It was a letter
to Bertrand Russell in which he agreed that his name should go on a
manifesto urging all nations to give up nuclear weapons. It is fitting that
one of his last acts was to argue, as he had done all his life, for
international peace.
Einstein was cremated at Trenton, New Jersey at 4 pm on 18 April 1955 (the
day of his death). His ashes were scattered at an undisclosed place.
Valutation
- Divide this biography into paragraphes and give them proper titles.
- Summarize in few lines the Einstein’s life.
- Was he only interested in Physics and science?
1. Mathematics: Lorentz’s transformations
teacher: Mr. Nicola Chiriano
As far as the laws of mathematics refer to reality, they are not
certain,
and as far as they are certain, they do not refer to reality.
We have already seen that Newtonian mechanics is
invariant under the
Galilean transformations relating two
inertial frames moving with relative
speed v in the x-direction,
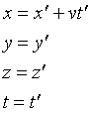
However, these transformations presuppose that time is a well-defined
universal concept, that is to say, it’s
the same time everywhere, and all observers can agree on what time it
is. Once we accept the basic postulate of special relativity, however, that
the laws of physics, including Maxwell’s equations, are the same in all
inertial frames of reference, and consequently the speed of light
has the same value in all inertial frames, then as we have seen, observers
in different frames do not agree on whether clocks some distance apart are synchronized.
Furthermore, as we have discussed, measurements of moving objects are
compressed in the direction of motion by the Lorentz-Fitzgerald contraction effect.
Obviously, the above equations are too naïve! We must think more carefully
about time and distance measurement, and construct new transformation
equations consistent with special relativity.
Our aim here, then, is to find a set of equations
analogous to those above giving the coordinates of an event (x,y,z,t) in
frame S, for example, a small bomb explosion, as functions of the
coordinates (x,y,z,t) of the same event measured in the parallel frame S’
which is moving at speed v along the x-axis of frame S. Observers O at the
origin in frame S and O’ at the origin in frame S synchronize their clocks
at t = t’ =0, at the instant they pass each other, that is, when the two
frames coincide.
Finally, we have found the Lorentz transformations expressing the
coordinates (x,y,z,t) of an event in frame S in terms of the coordinates (x’,y’,z’,t’)
of the same event in frame S’:
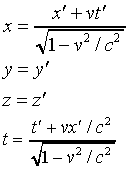
Notice that nothing in the above derivation depends on the x-velocity v
of S’ relative to S being positive. Therefore, the inverse transformation
(from (x,y,z,t) to (x’, y’, z’,t’)) has exactly the same form as
that given above with v replaced by -v.
Valutation
- Explain in few lines the meaning of the eight highlighted words. (8/10
points)
- Read Lorentz’s formulas. (2/10 points)
2. Physics – Special Relativity postulates and their
consequences
teacher: Mr. Nicola Chiriano
Put your hand on a hot stove for a minute, and it seems like an hour.
Sit with a pretty girl for an hour, and it seems like a minute. That’s
relativity.
Special Relativity: Postulates
• Principle of Relativity
All the laws of physics are the same in all inertial reference frames, i.e.
frames moving at constant velocities respect to each other.
• Constant Speed of Light
The speed of light, c = 3×108 m/s, is equal in all inertial
frames, regardless of the velocity of the observer or the light source.
• Consequences of Special relativity
– “Slowing” down of clocks (time dilation) and length contraction in
moving reference frames as measured by an observer in another reference
frame.
3. Science - The Atomic Bomb / Black Holes
teacher: Mrs. Maria Giuffrida
“Science without religion is lame, religion without science is blind.”
FISSION/CHAIN
REACTION
The atomic bomb gets it's energy from the fission (splitting) of
the nuclei (core) of uranium or plutonium atoms. Albert Einstein
explained how the fission of heavy atoms can produce energy released
as dangerously high levels of heat and radiation. He published his
theory in 1905 which is the well-known equation E = m c2.
This states that a given mass (m), is associated with an amount of
energy (E), equal to this mass multiplied by the square of the speed
of light (c).
A very small amount of matter is equivalent to a vast amount of
energy. For example, 1 kg of matter converted completely into energy
would be equivalent to the energy released by exploding 22 megatons of
TNT.
The neutron is the most effective particle to cause uranium fission.
Only one neutron is needed to split an atom. When the atom fissions
(splits), it splits into two smaller atoms which are most always
radioactive and releases an enormous amount of energy and two or three
neutrons. The neutrons released could then possibly hit other nuclei
of uranium which causes them to split in the same fashion. This is a
chain reaction (a series of fissions).
CRITICAL MASS
If you had a small sphere of pure fissile material, such as
uranium-235, about the size of a golf ball, it would not sustain a
chain reaction. Too many neutrons escape through the surface area, and
in turn are lost to the chain reaction. This is called a subcritical
amount.
In a mass of uranium-235 about the size of a baseball, there are more
neutrons hitting the atoms of the fissile material than are escaping
through the surface area, thus sustaining the chain reaction. The
minimum amount of fissile material required to maintain the chain
reaction is known as the critical mass.
Increasing the size of the sphere produces a supercritical assembly,
in which the successive generations of fissions increase very rapidly,
leading to a possible explosion as a result of the extremely rapid
release of a large amount of energy. A heavy material, called a
tamper, surrounds the fissile mass and prevents its premature
disruption. The tamper also reduces the number of neutrons that
escape.
PRODUCING AN EXPLOSION
When the scientist assemble the bomb, they cannot just create a
supercritical mass of fissile material because it would explode.
We get around this problem by creating two subcritical amounts of
fissile material then assembling them in the bomb apart from each
other.They do not become critical until an explosion is set off to
fire one of the subcritical masses at the other one. The force of the
impact welds the two pieces together. Together, these create a
critical mass.
It takes about 1 millionth of a second for the nuclear explosion to
occur.
THE MANHATTAN PROJECT
Research on atomic bombs was begun around the same time in several
countries, including Germany, but in the United States, the actual
building of an atomic bomb was already underway by 1942 under the code
name "Manhattan Project."
The project was carried out in extreme secrecy using a large amount of
the national budget. Many prominent American scientists including the
physicists Enrico Fermi and J. Robert Oppenheimer, and the chemist
Harold Urey, were associated with the project, which was headed by a
U.S. Army engineer, Major General Leslie Groves.
In September 1944 it was determined that an A-bomb would be used
against Japan.
On July 16, 1945 in the desert near Alamogordo, New Mexico, the United
States successfully conducted the world 's first nuclear test, the
"Fat Man" test, codename Trinity. The bomb used in the
Trinity test was called the "Fat Man".
When the bomb exploded and the fireball continued to consume the
desert, General Thomas F. Farrell, Groves' assistant cried out,
"the longhaors have let it get away from them!"
Black Holes
A
black hole is a region of space that has so much mass concentrated in
it that there is no way for a nearby object to escape its
gravitational pull. Since our best theory of gravity at the moment is
Einstein's general theory of relativity, we have to delve into some
results of this theory to understand black holes in detail, but let's
start of slow, by thinking about gravity under fairly simple
circumstances.
The idea of a mass concentration so dense that even light would be
trapped goes all the way back to Laplace in the 18th century. Almost
immediately after Einstein developed general relativity, Karl
Schwarzschild discovered a mathematical solution to the equations of
the theory that described such an object. It was only much later, with
the work of such people as Oppenheimer, Volkoff, and Snyder in the
1930's, that people thought seriously about the possibility that such
objects might actually exist in the Universe. (Yes, this is the same
Oppenheimer who ran the Manhattan Project.) These researchers showed
that when a sufficiently massive star runs out of fuel, it is unable
to support itself against its own gravitational pull, and it should
collapse into a black hole.
In general relativity, gravity is a manifestation of the curvature of
spacetime. Massive objects distort space and time, so that the usual
rules of geometry don't apply anymore. Near a black hole, this
distortion of space is extremely severe and causes black holes to have
some very strange properties. In particular, a black hole has
something called an 'event horizon.' This is a spherical surface that
marks the boundary of the black hole. You can pass in through the
horizon, but you can't get back out. In fact, once you've crossed the
horizon, you're doomed to move inexorably closer and closer to the
'singularity' at the center of the black hole. You can think of the
horizon as the place where the escape velocity equals the velocity of
light. Outside of the horizon, the escape velocity is less than the
speed of light.
The horizon has some very strange geometrical properties. To an
observer who is sitting still somewhere far away from the black hole,
the horizon seems to be a nice, static, unmoving spherical surface.
But once you get close to the horizon, you realize that it has a very
large velocity. In fact, it is moving outward at the speed of light!
That explains why it is easy to cross the horizon in the inward
direction, but impossible to get back out. Since the horizon is moving
out at the speed of light, in order to escape back across it, you
would have to travel faster than light. You can't go faster than
light, and so you can't escape from the black hole.
Incidentally, the name 'black hole' was invented by John Archibald
Wheeler, and seems to have stuck because it was much catchier than
previous names. Before Wheeler came along, these objects were often
referred to as 'frozen stars.' I'll explain why below.
A typical mass for such a stellar black hole would be about 10 times
the mass of the Sun, or about 1031 kilograms.Astronomers also suspect
that many galaxies harbor extremely massive black holes at their
centers. These are thought to weigh about a million times as much as
the Sun, or 1036 kilograms.
The more massive a black hole is, the more space it takes up. In fact,
the Schwarzschild radius (which means the radius of the horizon) and
the mass are directly proportional to one another: if one black hole
weighs ten times as much as another, its radius is ten times as large.
A black hole with a mass equal to that of the Sun would have a radius
of 3 kilometers. So a typical 10-solar-mass black hole would have a
radius of 30 kilometers, and a million-solar-mass black hole at the
center of a galaxy would have a radius of 3 million kilometers. Three
million kilometers may sound like a lot, but it's actually not so big
by astronomical standards. The Sun, for example, has a radius of about
700,000 kilometers, and so that supermassive black hole has a radius
only about four times bigger than the Sun.
A black hole has a "horizon," which means a region from
which you can't escape. If you cross the horizon, you're doomed to
eventually hit the singularity. But as long as you stay outside of the
horizon, you can avoid getting sucked in. In fact, to someone well
outside of the horizon, the gravitational field surrounding a black
hole is no different from the field surrounding any other object of
the same mass. Only stars that weigh considerably more than the Sun
end their lives as black holes. The Sun is going to stay roughly the
way it is for another five billion years or so. Then it will go
through a brief phase as a red giant star, during which time it will
expand to engulf the planets Mercury and Venus, and make life quite
uncomfortable on Earth (oceans boiling, atmosphere escaping, that sort
of thing). After that, the Sun will end its life by becoming a boring
white dwarf star.
What if the Sun did become a black hole for some reason? The main
effect is that it would get very dark and very cold around here. The
Earth and the other planets would not get sucked into the black hole;
they would keep on orbiting in exactly the same paths they follow
right now. Why? Because the horizon of this black hole would be very
small -- only about 3 kilometers -- and as we observed above, as long
as you stay well outside the horizon, a black hole's gravity is no
stronger than that of any other object of the same mass.
You can't see a black hole directly, of course, since light can't get
past the horizon. That means that we have to rely on indirect evidence
that black holes exist.
Suppose you have found a region of space where you think there might
be a black hole. How can you check whether there is one or not? The
first thing you'd like to do is measure how much mass there is in that
region. If you've found a large mass concentrated in a small volume,
and if the mass is dark, then it's a good guess that there's a black
hole there. There are two kinds of systems in which astronomers have
found such compact, massive, dark objects: the centers of galaxies
(including perhaps our own Milky Way Galaxy), and X-ray-emitting
binary systems in our own Galaxy.
These massive dark objects in galactic centers are thought to be black
holes for at least two reasons. First, it is hard to think of anything
else they could be: they are too dense and dark to be stars or
clusters of stars. Second, the only promising theory to explain the
enigmatic objects known as quasars and active galaxies postulates that
such galaxies have supermassive black holes at their cores. If this
theory is correct, then a large fraction of galaxies -- all the ones
that are now or used to be active galaxies -- must have supermassive
black holes at the center. Taken together, these arguments strongly
suggest that the cores of these galaxies contain black holes, but they
do not constitute absolute proof.
Two very recent discovery has been made that strongly support the
hypothesis that these systems do indeed contain black holes. First, a
nearby active galaxy was found to have a "water maser"
system (a very powerful source of microwave radiation) near its
nucleus. Using the technique of very-long-baseline interferometry, a
group of researchers was able to map the velocity distribution of the
gas with very fine resolution. In fact, they were able to measure the
velocity within less than half a light-year of the center of the
galaxy. From this measurement they can conclude that the massive
object at the center of this galaxy is less than half a light-year in
radius. It is hard to imagine anything other than a black hole that
could have so much mass concentrated in such a small volume.
A second discovery provides even more compelling evidence. X-ray
astronomers have detected a spectral line from one galactic nucleus
that indicates the presence of atoms near the nucleus that are moving
extremely fast (about 1/3 the speed of light). Furthermore, the
radiation from these atoms has been redshifted in just the manner one
would expect for radiation coming from near the horizon of a black
hole. These observations would be very difficult to explain in any
other way besides a black hole, and if they are verified, then the
hypothesis that some galaxies contain supermassive black holes at
their centers would be fairly secure.
QUESTIONNAIRE
1. How can you produce energy in the atomic bomb?
2. What's the critical mass?
3. How can an explosion be produced ?
4. Could you speak about the Manhattan Project?
5. Who were the famous American scientists involved in the project?
6. What is a black hole?
7. How big is a black hole?
8. If a black hole existed, would it suck up all the matter in the
Universe?
9. Is there any proof of the existence of the black holes?
10. What happened if the Sun became a black hole?
4. History: The Second World War - the position of
scientists
teacher: Mrs. Antonella Aletta
I don’t know with what weapons World War III will be fought,
but World War IV will be fought with sticks and stones.
"Concern
for man himself must always constitute the chief objective of all
technological effort -- concern for the big, unsolved problems of how to
organize human work and the distribution of commodities in such a manner as
to assure that the results of our scientific thinking may be a blessing to
mankind, and not a curse."
Scientists in the 1930s, using machines that could break apart the
nuclear cores of atoms, confirmed Einstein’s formula E=mc² . The release
of energy in a nuclear transformation was so great that it could cause a
detectable change in the mass of the nucleus. But the study of nuclei - in
those years the fastest growing area of physics - had scant effect on
Einstein. Nuclear physicists were gathering into ever-larger teams of
scientists and technicians, heavily funded by governments and foundations,
engaged in experiments using massive devices. Such work was alien to
Einstein’s habit of abstract thought, done alone or with a mathematical
assistant. In return, experimental nuclear physicists in the 1930s had
little need for Einstein’s theories.
In
August 1939 nuclear physicists came to Einstein, not for scientific but for
political help. The fission of the uranium nucleus had recently been
discovered. A long-time friend, Leo Szilard, and other physicists realized
that uranium might be used for enormously devastating bombs. They had reason
to fear that Nazi Germany might construct such weapons. Einstein, reacting
to the danger from Hitler’s aggression, had already abandoned his strict
pacifism. He now signed a letter that was delivered to the American
president, Franklin D. Roosevelt, warning him to take action. This letter,
and a second Einstein-Szilard letter of March 1940, joined efforts by other
scientists to prod the United States government into preparing for nuclear
warfare. Einstein played no other role in the nuclear bomb project. As a
German who had supported left-wing causes, he was denied security clearance
for such sensitive work. But during the war he did perform useful service as
a consultant for the United States Navy’s Bureau of Ordnance.
The photograph shows a postwar reconstruction of the signing.
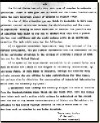
"Because of the danger that Hitler might be the first to have the bomb,
I signed a letter to the President which had been drafted by Szilard. Had I
known that the fear was not justified, I would not have participated in
opening this Pandora’s box, nor would Szilard. For my distrust of
governments was not limited to Germany."
Click on the picture to start PPS slides.
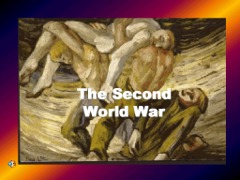
5. Philosophy: “The World as I see it”
teacher: Mrs. Antonella Aletta
Click on a picture to start PPS slides.
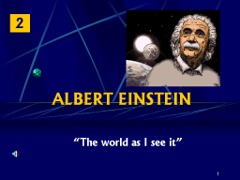
6. English Literature: The stream of consciousness
teacher: Mrs. Maria Simone
7. Arts: Munch and Dalì
The most beautiful thing we can experience is the mysterious.
It is the
source of all true art and science.
8. Religion: Einstein’s God
My religion consists of a humble admiration of the illimitable superior
spirit
who reveals himself in the slight details we are able to perceive with our
frail and feeble mind.
"The most beautiful experience we can have is the mysterious. It is
the fundamental emotion that stands at the cradle of true art and true
science. Whoever does not know it and can no longer wonder, no longer
marvel, is as good as dead, and his eyes are dimmed. It was the experience
of mystery -- even if mixed with fear -- that engendered religion. A
knowledge of the existence of something we cannot penetrate, our perceptions
of the profoundest reason and the most radiant beauty, which only in their
most primitive forms are accessible to our minds: it is this knowledge and
this emotion that constitute true religiosity. In this sense, and only this
sense, I am a deeply religious man... I am satisfied with the mystery of
life’s eternity and with a knowledge, a sense, of the marvelous structure
of existence -- as well as the humble attempt to understand even a tiny
portion of the Reason that manifests itself in nature."
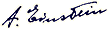
|